A redox reaction is one that involves an exchange of electrons between reactants.
Many chemical transformations involve the transfer of one or more electrons from one atom to another. These reactions, whether synthesis, decomposition, combustion or photosynthesis, all involve two simultaneous reactions: oxidation and reduction. Since a redox reaction involves both reactions at the same time, it is referred to as an oxidation half-reaction and a reduction half-reaction. It should be noted that, despite what its name might suggest, the presence of oxygen is not a prerequisite for oxidation. The operation of an electrochemical cell involves a redox reaction.
The formation of rust is an example of oxidation-reduction since it involves a transfer of electrons between iron and oxygen according to the following reaction:
|4 Fe_{(s)} + 3 O_{2(g)}| → |2 Fe_{2}O_{3(s)}|.
The image on the right represents the rust layer that develops on the surface of the iron as a result of contact with atmospheric oxygen.
During an oxidation half-reaction, an atom loses one or more of its electrons. Each time a substance gives up an electron, its ionic charge, which corresponds to its degree of oxidation, increases by 1.
In this half-reaction equation, the electrons are always components of the products; they are therefore located to the right of the arrow in the equation. The substance that donates one or more electrons, in this case the reactant in the reaction, is called the reducing agent. This substance can also be called the oxidised substance.
Here are some examples of oxidation half-reactions:
|Mg_{(s)} \rightarrow Mg^{2+}_{(aq)} + 2e^-|
|Cu^{1+}_{(aq)} \rightarrow Cu^{2+}_{(aq)} + 1e^-|
|Al_{(s)} \rightarrow Al^{3+}_{(aq)} + 3e^-|
In the examples given, the |Mg_{(s)}|, |Cu^{1+}_{(aq)}| and |Al_{(s)}| are both reducing agents and oxidised substances.
The degree of oxidation of a particle (atom, molecule or ion) corresponds to a charge (fictitious or arbitrary) that this element would have if the particle in which it is found were totally ionic. Thus, by convention, all the elements in the atomic state have a degree of oxidation corresponding to 0, such as |Mg_{(s)}|and |Al_{(s)}|in the previous examples.
According to the law of conservation of matter, electrons can neither be lost nor created. Therefore, another atom must accept the electrons from the oxidation half-reaction for it to occur.
During a reduction half-reaction, an atom gains one or more electrons. Each time a substance gains or accepts an electron, its degree of oxidation decreases by 1.
In this half-reaction equation, the electrons are always components of the reactants; they are therefore located to the left of the arrow in the equation. The substance that accepts electrons, in this case the reactants in the reaction, is called theoxidising agent. This substance can also be called the reduced substance.
Here are some examples of reduction half-reactions:
|Fe^{3+}_{(aq)} + 1e^- \rightarrow Fe^{2+}_{(aq)}|
|Cu^{1+}_{(aq)} + 1e^- \rightarrow Cu_{(s)}|
|Al^{3+}_{(aq)} + 3e^- \rightarrow Al_{(s)}|
In the examples given, |Fe^{3+}_{(aq)}|, |Cu^{1+}_{(aq)}| and |Al^{3+}_{(aq)}| are both oxidising agents and reduced substances.
According to the law of conservation of matter, electrons can neither be lost nor created. Therefore, the electrons accepted by each atom in the reduction half-reaction must come from another atom that donated them in the oxidation half-reaction.
An exchange of electrons between reactants always takes place in a redox reaction. The reactant that gains electrons is called the oxidant because it causes the oxidation of another substance. Conversely, the reactant that loses electrons is called the reductant because it causes the reduction of the other substance. In a redox reaction, the oxidant and the reductant form a torque linked by an exchange of electrons. The table below summarises the characteristics of the two types of actors involved in a redox reaction.
Agent | Electron exchange | Half-reaction | The atom is... |
Reducer | It loses electrons. | Oxidation | Oxidation |
Oxidation agent | It gains electrons. |
Reduction |
Reduced |
In a redox reaction, the oxidant and the reductant form a torque linked by an exchange of electrons.
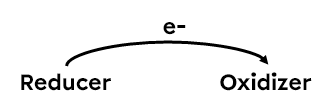
To obtain the overall oxidation-reduction equation, the two half-reactions, oxidation and reduction, are added together. For example, the redox reaction between solid aluminium and copper ions in aqueous solution involves the following two half-reactions:
Oxidation equation: |Al_{(s)} \rightarrow Al^{3+}_{(aq)} + 3e^-|
Reduction equation: |Cu^{1+}_{(aq)} + 1e^- \rightarrow Cu_{(s)}|
Before adding the half-reactions, we need to check that the number of electrons given is equal to the number of electrons received. In the example, the aluminium ion carries a charge of 3+ after oxidation, whereas the copper ion carries a charge of 1+. The reduction equation must therefore be multiplied by 3 to ensure that the number of electrons is equal in both the products of the oxidation reaction and the reactants in the reduction reaction.
After multiplying the reduction reaction by 3, we obtain the following two half-reactions:
Oxidation equation: |Al_{(s)} \rightarrow Al^{3+}_{(aq)} + 3e^-|
Reduction equation: |3 Cu^{1+}_{(aq)} + 3e^- \rightarrow 3 Cu_{(s)}|
The two equations can now be added together. It is important to note that the three electrons on either side of the arrow cancel each other out.
Global redox equation:
|Al_{(s)} + 3 Cu^{1+}_{(aq)} \rightarrow Al^{3+}_{(aq)} + 3 Cu_{(s)}|
This equilibrium exists since the sum of the charges (i.e. 0) is identical on both sides of the system.
In a redox equation, there is always a solid element and an ion in the aqueous phase involved, both in the reactants and in the products. In addition, the solid reactant becomes an ion in the products, while the ionic reactant becomes a solid element in the products.
Terminology
In relation to the example above, we can summarise the main terms used in a redox phenomenon.
Oxidation half-reaction: equation in which the electrons are to the right of the reaction arrow; this is the reaction involving solid aluminium in the example.
Reduction half-reaction: equation in which the electrons are to the left of the reaction arrow; this is the reaction involving copper ions in the example.
Reducing agent: this is the electron donor; it is solid aluminium in the example. The reducing agent increases the degree of oxidation and the substance is said to beoxidised.
Oxidising agent: this is the electron acceptor; in the example it is the copper ion. The oxidising agent reduces its degree of oxidation and the substance is said to be reduced.
The oxidation number, also known as the degree of oxidation, corresponds to the relative charge of an atom. It indicates the number of electrons gained or lost during the redox reaction.
The oxidation number is always a whole number. By convention, all elements in their fundamental state have an oxidation number of 0. It acquires a positive value when electrons are lost and becomes negative when electrons are gained. The oxidation number therefore increases during oxidation and, conversely, decreases during reduction. The sum of the oxidation numbers of the atoms that make up a molecule is zero. If it is not zero, we are in the presence of a polyatomic ion.
Certain rules can be used to determine the oxidation number of an atom:
- The oxidation number of a simple element is 0.
- The oxidation number of a monatomic ion corresponds to the charge of the ion.
- The oxidation number of oxygen is -2 except in the case of a peroxide where it is -1.
- The oxidation number for hydrogen is generally +1, except in cases where the hydrogen is bonded solely to a metal (in which case it is -1).
- The oxidation number of a metal is always positive, whereas that of a non-metal can be positive or negative.
- The most likely oxidation number for an element depends on the number of valence electrons in the family to which the element belongs.
- The sum of the oxidation numbers of a molecule is zero.
- The sum of the oxidation numbers of a polyatomic ion is equal to the charge of that ion.
The reduction potential, or E°, is the relative capacity of an element to accept electrons. It is evaluated by comparison with the ion |H^{+}_{(aq)}| in the following reduction half-reaction: |2 H^+_{(aq)} + 2 e^- \rightleftharpoons H_{2(g)}|.
Each element, whether ion, atom or molecule, has a certain capacity to receive electrons. The potential of an element to receive one or more electrons is measured in volts (V). The value for hydrogen has been arbitrarily set at 0.00 V. All the elements that are better electron acceptors (or better oxidisers) than |H^{+}_{(aq)}| will have a reduction potential greater than that of hydrogen (Eº > 0.00 V). On the other hand, all those with weaker electrons will have a reduction potential lower than that of hydrogen (Eº < 0.00 V).
By carrying out research phases at a temperature of 25ºC, a pressure of 101.3 kPa and with ionic solutions at a concentration of 1 mol/L, standard reduction potentials were determined for many substances. Some of these are listed in the following link:
The reducing power of a metal defines its tendency to give up electrons and oxidise.
A spontaneous oxidation-reduction reaction occurs when this happens naturally, without any external energy input.
The tendency to donate electrons varies from one metal to another. In general, alkali metals and alkaline earth metals are considered to be good donors. Metals can be classified according to their reducing power.

Using this classification, we can predict whether a reaction occurs spontaneously or requires an input of energy. For a spontaneous redox reaction to occur between two metals, the stronger reductant must be in a solid state and the weaker one must be in the form of ions in an aqueous environment. The conditions necessary for a spontaneous reaction to take place are therefore as follows:
Reaction |
Strongest reducer |
Lowest reduction ratio |
Spontaneous | Solid | Aqueous solution |
No | Aqueous solution | Solid |
Thus, solid aluminium in the presence of copper ions would produce a spontaneous reaction, whereas the reverse reaction, solid copper in the presence of aluminium ions, would require an input of energy to take place.
The possibility of a spontaneous reaction can also be verified by adding the values of the reduction potentials Eº of the two half-reactions involving the reactants.
- If the total energy value (Eº) is positive, then the reaction will be spontaneous.
- Otherwise (≤ 0.00 V), the reaction will not be possible, as the reverse reaction will be favoured.
Understanding the spontaneity of a reaction is fundamental in chemistry, as it is the basic or alkaline principle on which electrochemical batteries are based.
Is it possible to keep a solution of |Cu^{2+}_{(aq)}| in a solid aluminium (Al) container?
1. Using the table of standard reduction potentials, determine the value of the Eº for each of the half-reactions.
|Cu^{2+}_{(aq)} + 2 e^- \rightarrow Cu_{(s)}| E° = + 0,34 V
|Al_{(s)} \rightarrow Al^{3+}_{(aq)} + 3 e^-| E° = + 1,66 V
We change the - sign in front of 1.66 V to + 1.66 V, because we need to invert the partial equation involving the |Al_{(s)}| in order to obey the desired reaction. Here |Cu^{2+}_{(aq)}| and |Al_{(s)}| are the reactants.
2. We must ensure that the total number of electrons exchanged is respected. The result is
|3 Cu^{2+}_{(aq)} + 6 e^-| → |3 Cu_{(s)}| E° = + 0,34 V
|2 Al_{(s)}| → |2 Al^{3+}_{(aq)} + 6 e^-| E° = + 1,66 V
Please note! Reduction potentials are not affected when equations are multiplied by a constant.
3. Now we need to determine the global equation.
|2 Al_{(s)} + 3 Cu^{2+}_{(aq)}| → |2 Al^{3+}_{(aq)} + 3 Cu_{(s)}|
E° total = + 2,00 V
4. There will be a spontaneous reaction, because the total Eº is positive, so it will be impossible to keep a solution of |Cu^{2+}_{(aq)}| in a container of Al.
One of the most important applications of a spontaneous reaction is the electrochemical cell. According to the law of conservation of energy, an electrochemical cell spontaneously transforms chemical energy into electrical energy.
The electrochemical cell is made up of two electrodes, called half-cells, each consisting of a metal part immersed in an ionic solution. The two electrodes are connected by a wire, while the two solutions are connected by a salt bridge, i.e. a tube filled with a solution of spectator ions that do not take part in the reaction. It is the exchange of charges between the two half-cells (electric intensity) and the ions in the solution that enables the electrochemical cell to convert between the two forms of energy.
The zinc-copper cell is a classic example of an electrochemical cell. Here is the diagram for this electrochemical cell:

Note: English image coming soon!
We will use a battery involving the spontaneous reaction occurring between electrodes of Zn in solution (|ZnSO_{4}|) and Cu in solution (|CuSO_{4}|).
An electrode is usually an electrically conductive rod, wire or plate that allows the exchange of electric charges both in the external circuit and in the solution. The electrode that generates the electrons is called the anode and the electrode that receives the electrons is called the cathode.
To determine which electrode will receive the electrons, we need to decide which of the two half-reactions involving Zn and Cu in solution is the better electron recipient or oxidiser. To do this, the Eº values are consulted using the standard reduction potential table.
It should be noted that the presence of |SO^{2-}_{4(aq)}| ions is not taken into account, as they are considered to be spectator ions. This means that these ions have no role to play in the oxidation-reduction reaction. In this reaction, Cu ions have a charge of 2+ because the charge of |SO_{4}| ions is always 2-.
|Cu^{2+}_{(aq)} + 2 e^-| → |Cu_{(s)}| E° = + 0,34 V
|Zn^{2+}_{(aq)} + 2 e^-| → |Zn_{(s)}| E° = - 0,76 V
Note that the Cu^{2+}_{(aq)}| ions have a higher Eº than the Zn^{2+}_{(aq)}| ions. Electrons will therefore be captured by the Cu electrode and supplied by the Zn electrode. The Zn electrode is therefore the anode, while the Cu electrode is the cathode.
In an electrochemical cell, the ions travelled from one electrode to the other. They need to be slowed down so that the duration of the chemical reaction (and therefore the operating time of the battery) is reasonable. We therefore use a salt bridge containing an electrolyte solution such as |KCl| or |KNO_{3}|. The choice of the type of salt bridge favours the use of spectator ions to avoid creating undesirable secondary reactions.
The ions present in the salt bridge act as a sort of towing device for the other ions. The negative ions, or anions (in this case the |SO_{4}^{2-}|), will move towards the anode, while the positive ions, or cations, will move towards the cathode (in this case the |Cu^{2+}| and |Zn^{2+}| ions). The salt bridge can be replaced by a porous wall made of a compound that allows the ions in solution to travelled while preventing the reaction from occurring too quickly.
A voltmeter is used to measure the potential difference (ddp) or voltage, expressed in volts, between the two half-cells. The direction of travelled of the voltmeter pointer corresponds to the direction of travelled distance of the electrons in the battery. An ammeter is used to measure the current in the battery.
It is possible to predict the voltage (or ddp) of an electrochemical cell. In the previous example, that of the cell, we can base ourselves on the table of standard reduction potentials using the reduction half-reactions:
|Cu^{2+}_{(aq)} + 2 e^- \rightarrow Cu_{(s)}| E° = + 0,34 V
|Zn^{2+}_{(aq)} + 2 e^- \rightarrow Zn_{(s)}| E° = - 0,76 V
Note that the |Cu^{2+}_{(aq)}| is a better electron acceptor than the |Zn^{2+}_{(aq)}|, so we need to reverse the equation for the weaker Eº, i.e. the one involving the |Zn^{2+}_{(aq)}|. The two half-reactions can then be added together:
|Cu^{2+}_{(aq)} + 2 e^- \rightarrow Cu_{(s)}| E° = + 0,34 V
|Zn_{(s)} \rightarrow Zn^{2+}_{(aq)} + 2 e^-| E° = + 0,76 V
Global redox equation:
|Zn_{(s)} + Cu^{2+}_{(aq)} \rightarrow Zn^{2+}_{(aq)} + Cu_{(s)}|
E°total = + 1,10 V