Three important principles describe the behaviour of fluids.
Archimedes' principle states that an object immersed in a fluid experiences an upward acting force equal to the weight of the fluid moved by the object.
When an object is immersed in a fluid, it moves a quantity of that fluid. If we immerse a golf ball in a glass of water, the golf ball occupies the place of a certain quantity of water. The water level in the glass rises as a result of the water being moved.
The pressure exerted by the fluid generates an upward force called the Archimedean buoyant force. The magnitude of this force is equal to the weight of the amount of water moved. This force tends to push the object upwards. On the other hand, the weight of the object, also called gravitational force, is a force that tends to push the object downward. Depending on the magnitude of the upward acting force and the weight of the object, the object can sink, stay at the same depth, or float.
Here are the three possible situations.
The weight of the object is greater than the weight of the fluid it is moving (upward acting force, or buoyant force). The downward force is therefore greater than the upward force. The resultant force is directed downward and the object sinks to the bottom.
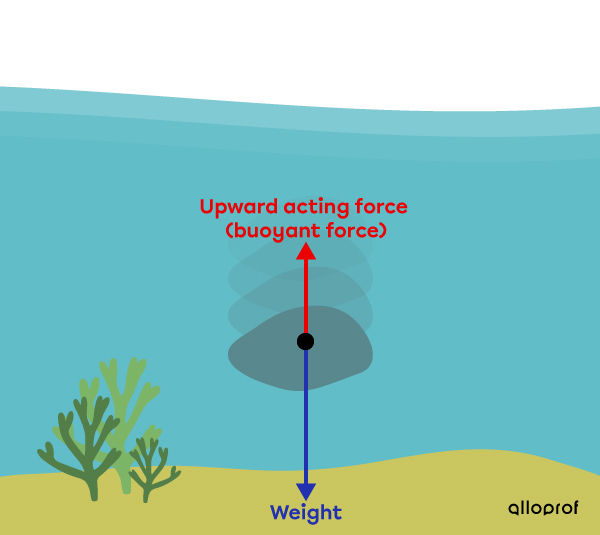
The weight of the object is equal to the weight of the fluid it is moving (buoyant force). The downward force and the upward force are of the same magnitude. They cancel each other out and the object remains at the depth where it is.
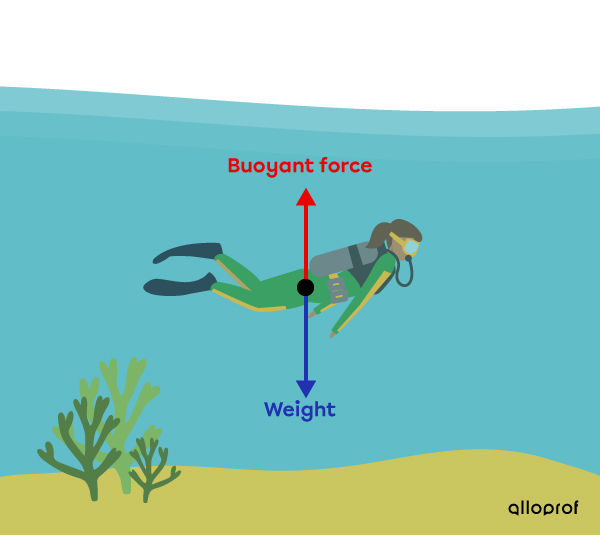
The weight of the object is less than the weight of the fluid it is moving (buoyant force). The downward force is smaller than the upward force. The resultant force is directed upward and the object surfaces.

If we put a ball of modelling clay in the water, it sinks. The volume of water it moves is not large enough to generate buoyant force greater than its weight.
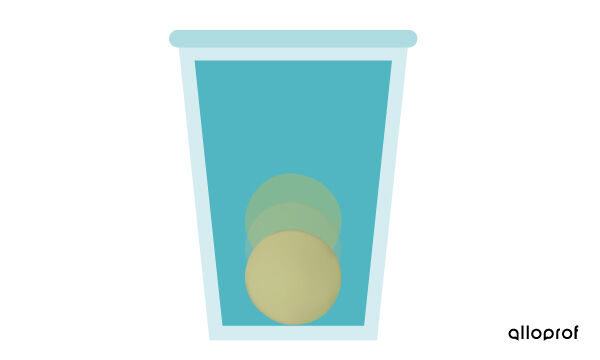
If we take the same ball of modelling clay, but shape it into a boat hull, it will float. In this form, the modelling clay moves a greater volume of water. The weight of the volume of water moved is therefore greater. The buoyant force is greater than the weight of the modelling clay, which remains the same.

The fact that a helium balloon floats in the air is also explained by Archimedes' principle.
The weight of the object is the weight of the balloon and the helium it contains.
The buoyant force applied to the balloon is the weight of the air that the balloon moves.
The weight of the helium-filled balloon is less than that of the air it moves. The weight is therefore smaller than the buoyant force. The resultant force is upwards and the balloon rises into the air.
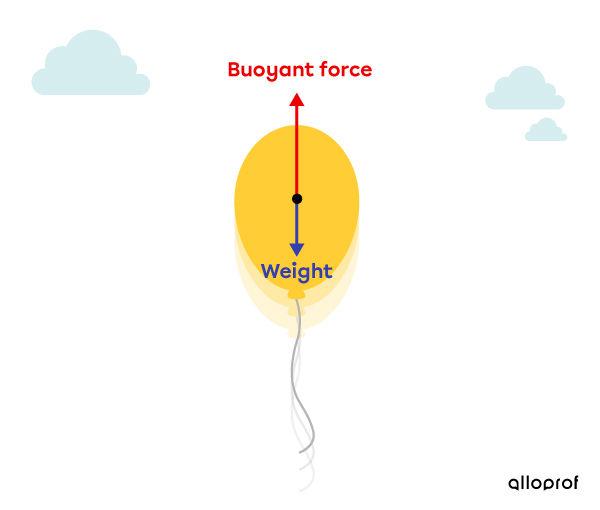
Pascal's principle states that a variation in pressure applied at a point in a fluid in a closed environment is distributed uniformly in all directions.
When a ball is inflated, the pressure at the entrance is increased. The air is distributed evenly in the ball. The pressure exerted by the air on the ball wall will then be equal in all directions.
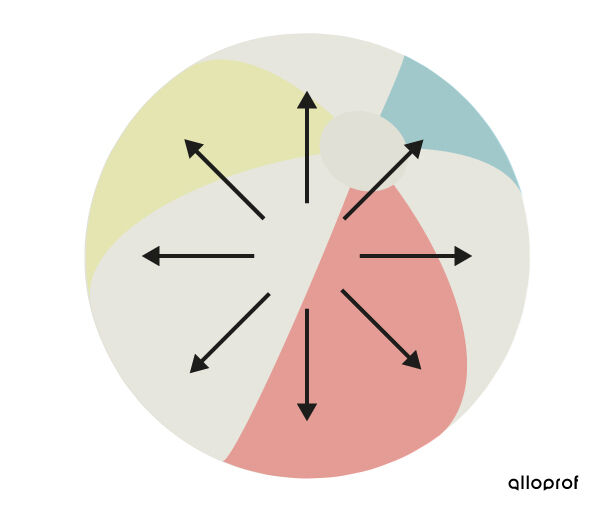
Hydraulic cylinders use Pascal's principle. These mechanisms are used to lift large loads, such as cars.
They consist of two pistons: a small surface piston and a large surface piston. These two pistons are interconnected and filled with an incompressible fluid, such as oil.
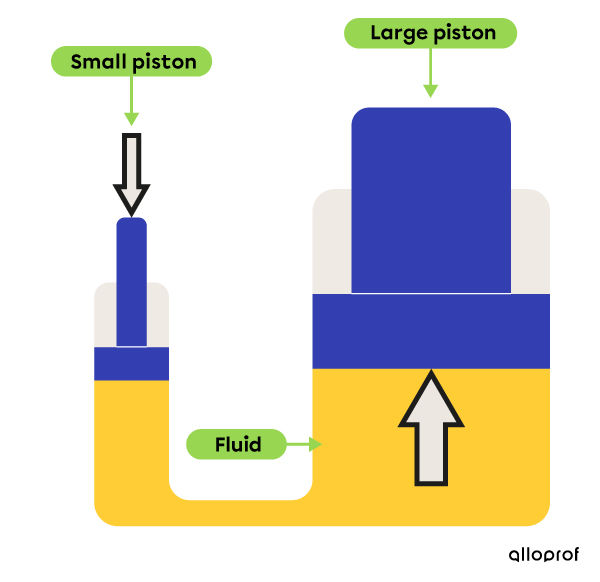
By pressing on the small piston, the pressure at a point in the cylinder fluid is increased. The pressure increase is then distributed evenly throughout the fluid. Thus, the increase in pressure of the large piston |(P_2)| is equal to that of the small piston |(P_1).|
||P_1=P_2||
It is also known that the pressure is equal to the ratio of the force applied to the area of the surface.
||P=\dfrac{F}{A}||
We can therefore say that |\dfrac{F_1}{A_1}=\dfrac{F_2}{A_2}.|
The difference in surface area of the pistons means that if a small force is applied to the small piston, a large force is generated by the large piston.
The small piston of a cylinder has a surface area of |0.002\ \text{m}^2,| while the large piston has a surface area of |0.010\ \text{m}^2.| If we apply a force of |100\ \text{N}| on the small piston, what force will be generated by the large piston?
The first step consists in identifying the data.
Small piston:
|\begin{align}&A_1=0.002\ \text{m}^2 &F_1=100\ \text{N}\end{align}|
Large piston:
|\begin{align}&A_2=0.010\ \text{m}^2 &F_2= ?\end{align}|
As |P_1=P_2| and |P=\dfrac{F}{A},| we can say that |\dfrac{F_1}{A_1}=\dfrac{F_2}{A_2}.|
|\begin{align}\dfrac{F_1}{A_1}=\dfrac{F_2}{A_2}\Rightarrow F_2&=\dfrac{F_1 \times A_2}{A_1}\\F_2&=\dfrac{100\ \text{N}\times{0.010\ \cancel{ \text{m}^2}}}{0.002\ \cancel{\text{m}^2}}\\F_2&=500\ \text{N} \end{align}|
The force generated by the large piston is |500\ \text{N}.| This force is indeed greater than that applied to the small piston |(100\ \text{N}).|
Bernoulli's principle states that the greater the speed of a fluid, the lower its pressure.
One method of observing this principle is to place a sheet of paper under the mouth. At rest, the paper falls.
When blowing straight ahead, the paper rises.
The air blown above the sheet of paper has a higher velocity than the air under the sheet. The air pressure above is therefore lower than that below. The paper is drawn towards the low pressure area, therefore upwards.
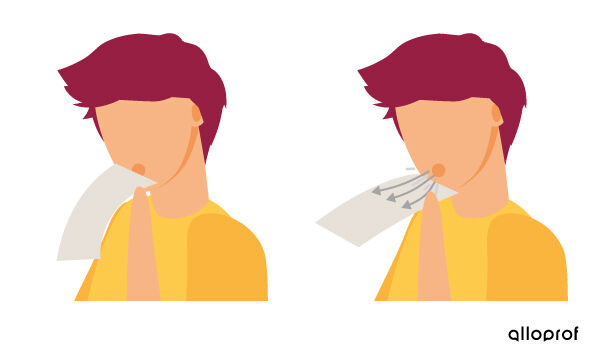
The operation of an airplane wing can be explained using Bernoulli's principle. The inclination and shape of the profile of an airplane wing deflects the path of air in such a way that the air above the wing has a greater velocity than the air below the wing. The high air velocity above the wing creates a low pressure area, while the low air velocity below the wing produces a high pressure area.
This pressure difference causes an upward force called lift. It allows the airplane to fly.
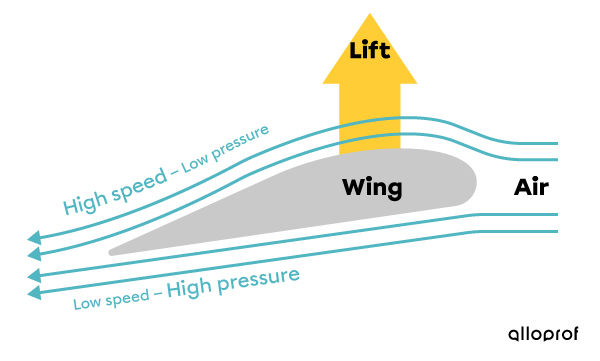