-
A catalyst is a substance that increases the rate of a chemical reaction by decreasing its activation energy without being consumed in the process.
-
Catalysis is the process of increasing the reaction rate with the help of a catalyst.
One of the principles of collision theory dictates that only particles with kinetic energy equal to or higher than the activation energy of a given chemical reaction can undergo successful collisions. A catalyst changes the reaction mechanism, which leads to a different orientation of the reactant molecules and increases the frequency of collisions. As a result, the activation energy required for the successful transformation of reactants into products decreases.
The following graph compares the energy diagram of a chemical reaction with and without a catalyst. The activation energy in the catalyzed reaction |(E_{a_{cat}})| is noticeably lower than the original activation energy |(E_a).| As the activation energy decreases, the number of successful collisions and the reaction rate both increase.
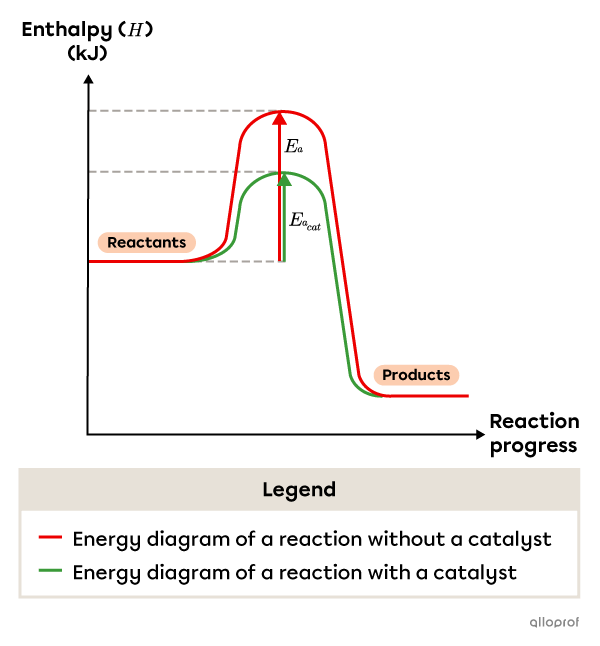
For instance, a two-step reaction mechanism can become a four-step reaction mechanism when a catalyst is added. Overall, the catalyzed reaction mechanism has a lower activation energy than the uncatalyzed reaction mechanism.
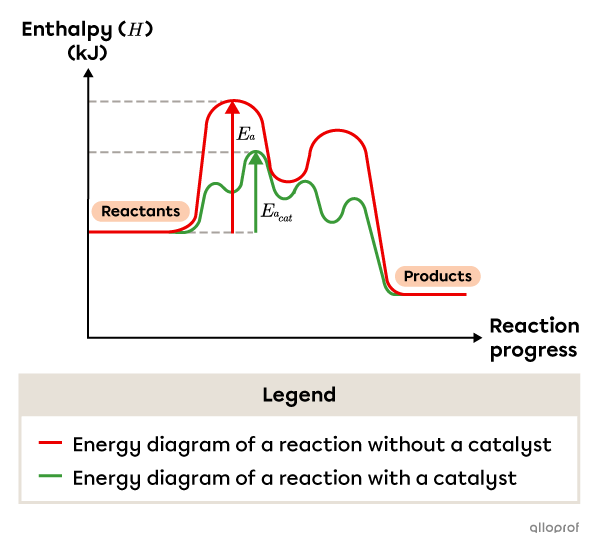
As a result of the lowered activation energy, the number of particles with sufficient kinetic energy for a successful reaction increases, as shown in the following Maxwell-Boltzman distribution.
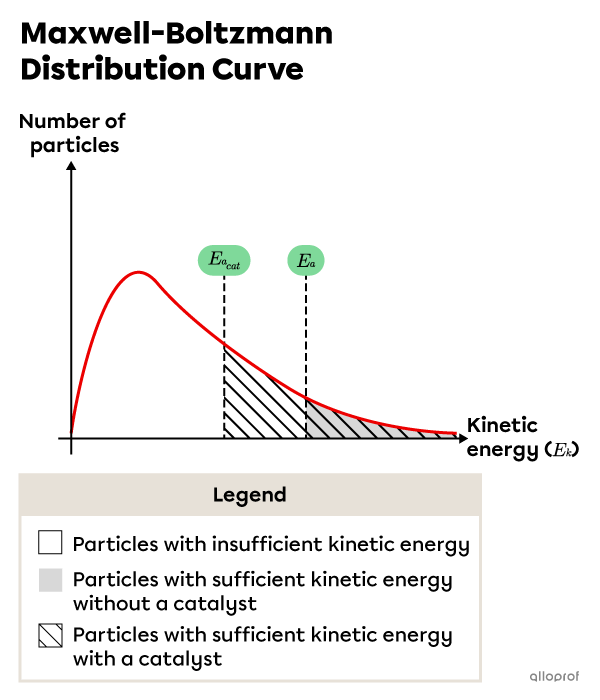
Particles in the unshaded region have less kinetic energy than the required activation energy. Particles in the shaded area have as much or more kinetic energy as the required activation energy without a catalyst. Particles in the lined area have as much or more kinetic energy as the required activation energy with a catalyst.
There are several important principles to understand about catalysts:
- Catalysts do not change the overall reaction equation: the chemical formula of the reactants and the desired products is not affected by the use of a catalyst.
Overall chemical equation without a catalyst: ||\text{A} + \text{B} \rightarrow \text{C}||
Overall chemical equation with a catalyst: ||\text{A} + \text{B} + \cancel{\text{Catalyst}} → C + \cancel{\text{Catalyst}}\\\\ \Rightarrow \text{A} + \text{B} \rightarrow \text{C}||
An alternative way frequently used to indicate the use of a catalyst is to write the word catalyst or the chemical formula of the substance used as a catalyst above the arrow in the chemical equation: ||A + B \xrightarrow{\text{Catalyst}} C|| - Catalysts do not affect the overall enthalpy change of the reaction:
||\Delta H = \Delta H_{(cat)}|| - Catalysts change the reaction mechanism, which leads to a change in the rate constant |(k)| as well as the rate law.
- Catalysts lower the activation energy of both the direct and inverse reactions, which means that both reaction rates are increased.
- Catalysts are reformed and can be recovered at the end of the reaction to be used again.
- Catalysts do not affect the position of the equilibrium of a chemical reaction but can ensure that it is attained sooner.
- Substances called inhibitors have the opposite effect of catalysts: they decrease the reaction rate.
Catalysts are commonly used in many industries to speed up important chemical reactions in a cost-efficient and environmentally conscious way. Using a catalyst can:
- decrease the amount of energy necessary to carry out a reaction;
- decrease the amount of chemical waste generated by the reaction, including greenhouse gases and other pollutants;
- make a reaction more efficient by increasing the amount of reactants effectively transformed into the desired products, which reduces the required initial amount of reactants.
Catalytic processes are applied in the production of many chemical substances and synthetic materials, the energy sector, pharmaceutical and medical research, food science, among others.
Aspirin, or acetylsalicylic acid |(\text{CH}_3\text{COOC}_6\text{H}_4\text{COOH}),| is synthesized from two reactants: salicylic acid |(\text{HOC}_6\text{H}_4\text{COOH})| and acetic anhydride |(\text{CH}_3\text{COOCOCH}_3).| This reaction is catalyzed by sulfuric acid |(\text{H}_2\text{SO}_4)\!:|
||\text{HOC}_6\text{H}_4\text{COOH} + \text{CH}_3\text{COOCOCH}_{3} \xrightarrow{\text{H}_2\text{SO}_4} \text{CH}_3\text{COOC}_6\text{H}_4\text{COOH} + \text{CH}_3\text{COOH}||
Without a catalyst, the activation energy of this reaction is too high and its rate is very slow. What's more, some of the reactants are wasted by not reacting or reacting to form unwanted products.
With a catalyst, the reaction uses a mechanism that allows a large portion of the reactants to be transformed into the desired products. Due to the lowered activation energy, the reaction can occur faster and at a lower temperature.
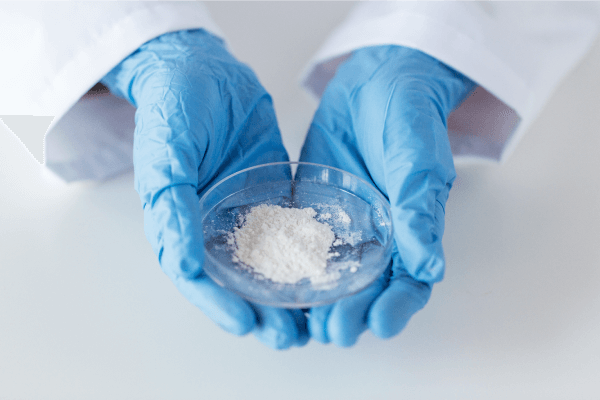
Ground Picture, Shutterstock
Catalysts are commonly classified as heterogeneous and homogeneous. Homogeneous catalysts include biological catalysts, also called enzymes.
A heterogeneous catalyst is a catalyst that is used in a state different from the state of the reactants.
Heterogeneous catalysts are usually metals or metal-based compounds in solid state. They are generally used to catalyze reactions where the reactants are in liquid, aqueous or gaseous state. Catalysis by heterogeneous catalysts is common in many fields, including production of chemicals, wastewater treatment, transformation of automotive pollutants and certain organic reactions.
The Haber Process is a catalytic process that uses a solid iron-based heterogeneous catalyst in the production of ammonia |(\text{NH}_3)| from hydrogen gas |(\text{H}_2)| and atmospheric nitrogen gas |(\text{N}_2)\!:|
||3\ \text{H}_{2\text{(g)}} + \text{N}_{2\text{(g)}} \rightarrow 2\ \text{NH}_{3\text{(g)}}||
The iron-based catalyst is a heterogeneous catalyst because it is in the solid state, whereas the reactants are in the gaseous state. The use of the heterogeneous catalyst results in an industrially significant amount of ammonia produced quickly. The catalyst can be continuously reused for the same reaction.
Consider this simplified diagram of the Haber Process:
-
Hydrogen |(\text{H}_2)| and nitrogen |(\text{N}_2)| gases are supplied into the reactor.
-
The catalyst inside a reactor provides a surface for hydrogen molecules and nitrogen molecules to be absorbed.
-
The catalyst weakens the chemical bonds within the molecules allowing them to separate into their respective atoms. The atoms diffuse along the surface of the catalyst.
-
New bonds form between some of the |(\text{H})| and |(\text{N})| atoms and ammonia molecules |(\text{NH}_3)| form. Ammonia gas is released from the surface of the catalyst.
-
The unreacted hydrogen |(\text{H}_2)| and nitrogen |(\text{N}_2)| gases are collected and used as reactants again.
-
The ammonia gas is condensed into liquid ammonia by the condenser.
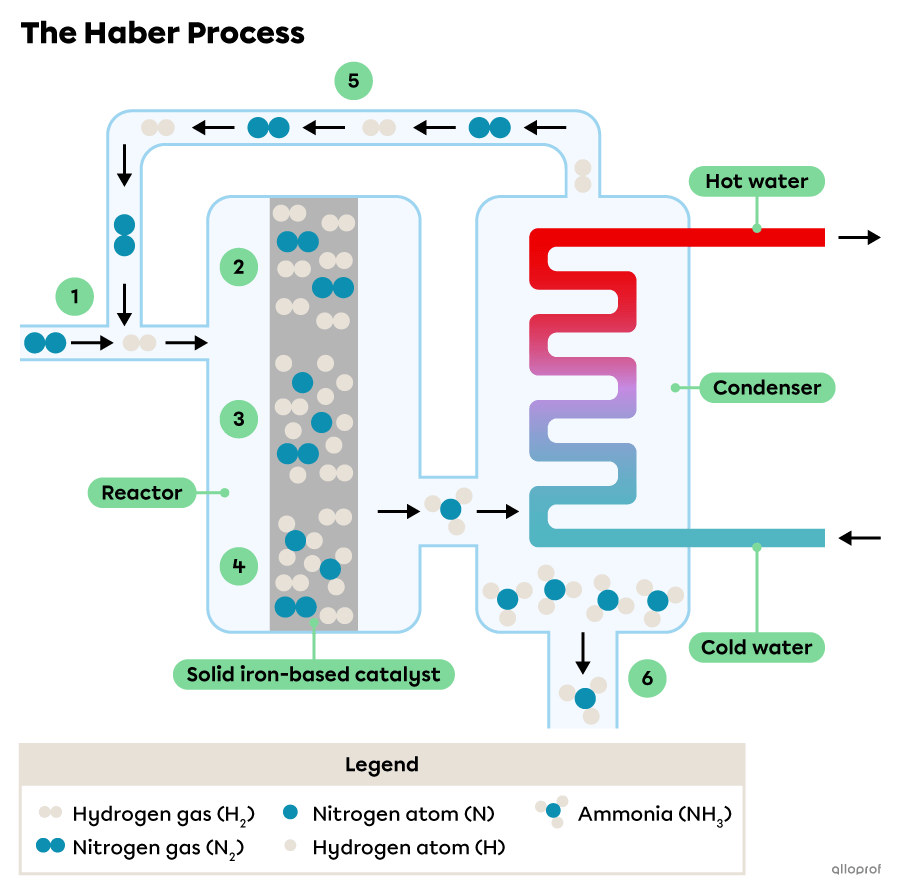
A homogeneous catalyst is a catalyst that is used in the same state as the state of the reactants.
Homogeneous catalysts generally catalyze reactions where the reactants are in solution. Catalysis by homogeneous catalysts is used in many fields, including pharmaceutical and medical research, biofuel development and pollutant degradation.
The thinning of the ozone layer |(\text{O}_3)| in the atmosphere is catalyzed by the presence of monatomic chlorine |(\text{Cl})| in the gaseous state. Since monatomic chlorine and ozone are both in the gaseous state, monatomic chlorine is a homogeneous catalyst. The catalyzed reaction mechanism passes by a reaction intermediate as follows:
Step 1: ||O_{3\text{(g)}} + \color{#EC0000}{Cl_{\text{(g)}}} \rightarrow O_{2\text{(g)}} + \color{#333FB1}{ClO_{\text{(g)}}}||
Step 2: ||O_{3\text{(g)}} + \color{#333FB1}{ClO_{\text{(g)}}} \rightarrow 2\ O_{2\text{(g)}} + \color{#EC0000}{Cl_{\text{(g)}}}||
Overall reaction: ||2\ O_{3\text{(g)}} + \cancel{\color{#EC0000}{Cl_{\text{(g)}}}} + \cancel{\color{#333FB1}{ClO_{\text{(g)}}}} \rightarrow O_{2\text{(g)}} + \cancel{\color{#333FB1}{ClO_{\text{(g)}}}} + 2\ O_{2\text{(g)}} + \cancel{\color{#EC0000}{Cl_{\text{(g)}}}} \\\\ ⇒ 2\ O_{3\text{(g)}} \rightarrow 3\ O_{2\text{(g)}}||
Note that the catalyst |(\color{#EC0000}{Cl_{\text{(g)}}})| can be identified because it is consumed in Step 1 and regenerated in Step 2. It is absent from the overall chemical equation.
Note that the reaction intermediate |(\color{#333FB1}{ClO_{\text{(g)}}})| can be identified because it forms at the end of Step 1 and is consumed during Step 2. It is absent from the overall chemical equation.
An enzyme, also called a biocatalyst, is a large protein that catalyzes a specific biochemical reaction.
Enzymes are highly specific proteins that accelerate biochemical reactions in living organisms. As homogeneous catalysts, they are involved in digestive processes, DNA replication, photosynthesis, the immune response and almost all other processes required for the survival of an organism. It is estimated that human cells contain over 3000 types of enzymes, each catalyzing a specific chemical reaction.
Several models have been developed in an attempt to explain how enzymes work. For example, the lock-and-key model proposes that the specificity of an enzyme is ensured by a specific location on the enzymatic molecule, called an active site, with a unique shape and chemical properties. Each active site is suitable for a specific reactant or group of reactants, called a substrate. Enzymes are sensitive to substrate concentration, as well as to the temperature and pH of the environment.
Enzymes are synthesized to be used in medical treatments in a variety of conditions. Additionally, inhibitors can be used to slow down enzymatic activity naturally occurring in the body. Newer enzyme applications, such as plastic biodegradation are studied by scientists.
The enzyme called lactase catalyzes the digestion of lactose, which is a sugar found in many dairy products. Lactose is the substrate of lactase. Normally, lactase produced in the small intestine breaks lactose into two simple sugars: galactose and glucose.
Mutations can lead to the production of defective lactase molecules and decreased gene activity in adults can result in less lactase in the digestive system. As a result, lactose cannot undergo chemical digestion and a condition known as lactose intolerance can develop. Based on the recommendation from a medical professional, lactase supplements can be ingested to catalyze lactose digestion.
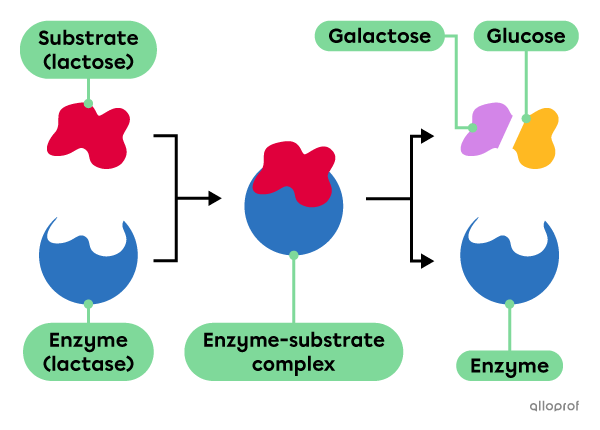
Extremophiles are microorganisms that are adapted to thrive in harsh environments such as extreme pH, temperature, salinity, pressure, radiation, oxidative stress, heavy metals, toxic chemicals and absence of water. In other words, their enzymes, known as extremozymes, are able to catalyze chemical reactions under unique extreme conditions. Researchers are interested in using extremozymes in harsh industrial processes and in the food industry, in order to increase important reaction rates and reduce production costs.
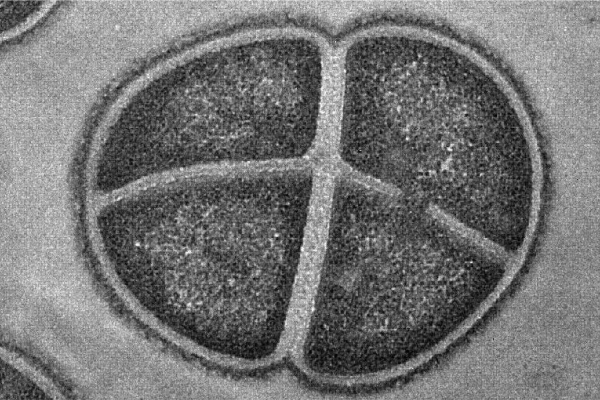
Deinococcus radiodurans is an extremophile bacterium studied by astrobiologists due to its resistance to extreme levels of ionizing radiation, including UV and gamma rays, as well as extremely dry, cold and acidic conditions. In fact, according to recent research, samples of Deinococcus radiodurans survived after being placed outside the International Space Station for 1 year[1].
Source: Life in the Extreme: Radiation [Online image]. Astrobiology at NASA, (2023, February 10). Rights reserved*[2]
- Ott, E., Kawaguchi, Y., Kölbl, D., Rabbow, E., Rettberg, P., Mora, M., Moissl-Eichinger, C., Weckwerth, W., Yamagishi, A., & Milojevic, T. (2020). Molecular repertoire of Deinococcus radiodurans after 1 year of exposure outside the International Space Station within the Tanpopo mission. Microbiome, 8(150). https://doi.org/10.1186/s40168-020-00927-5
- Life in the Extreme: Radiation [Online image]. Astrobiology at NASA, (2023, February 10). https://astrobiology.nasa.gov/news/life-in-the-extreme-radiation/ *Content used by Alloprof in compliance with the Copyright Act in the context of fair use for educational purposes. [https://laws-lois.justice.gc.ca/eng/acts/c-42/page-9.html].